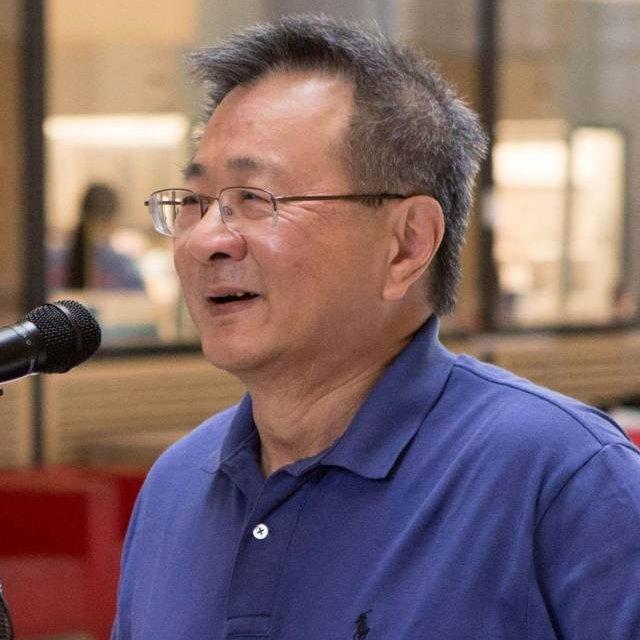
Our lab specializes in transport experiments on topological and strongly correlated phases of matter. We conduct electrical measurements of bulk crystals and nanofabricated devices made from exfoliated flakes. We also specialize in sensitive measurements of the thermal transport in insulators, including the thermal Hall effect.
Dirac/Weyl semimetals
In recent years, our group has uncovered a number of unusual transport features in topological semimetals. These materials have Dirac cones with nodes that are protected against hybridization by crystalline symmetry. In an applied magnetic field, each Dirac node becomes a superposition of Weyl nodes. (Weyl states are closely similar to massless Dirac states except they have an intrinsic handedness called chirality. In k space, Weyl nodes act as monopole sources and sinks of Berry curvature). A few years ago, our group engaged heavily in understanding how the chiral anomaly arises in these systems. Now, we are more focused on nontrivial transport effects involving topological surface states such as those that arise in very thin, micro-structured devices.
Thermal Hall effect
We probe the physics of systems with charge-neutral excitations using thermal conductivity and thermal Hall effect measurements in strong magnetic fields. The thermal Hall effect is the heat transport analog of the electrical Hall effect. One of the themes investigated is the notion that neutral currents (e.g. phonons and spin waves) can exhibit a large thermal Hall effect even though there is no Lorentz force. Again the origin is the Berry curvature. Recently, we discovered robust oscillations (periodic in 1/B) in the thermal conductivity of the quantum spin liquid candidate α-RuCl3, which may indicate the presence of a Fermi surface of quasiparticles that are fractionalized by strong entanglement.
Superconductivity of topological materials
We study various aspects of superconductivity in topological materials. Some superconducting topological materials, such as MoTe2, host intrinsic edge supercurrents. Using various transport techniques, we study the nature of these edge supercurrents, and we use them to probe interaction between multiple superconducting condensates in hybrid systems. Utilizing advanced nanofabrication facilities, our lab also creates various types of superconducting devices with topological materials, including asymmetric SQUIDs (superconducting quantum interference devices). By carefully tuning the phases of these devices, we investigate the current-phase relations of the superconducting junction of interest, searching for signatures of the anomalous 4π-periodic Josephson effect that is predicted to be found in topological superconducting junctions.
Two-dimensional materials
We are studying the quantum Hall effect in graphene as well as unconventional superconductivity in the 2D limit. We are developing the know-how to fabricate ultrahigh mobility graphene devices and manipulate quantum Hall edge modes. We are also developing devices to measure the thermopower and Nernst effect in 2D superconductors. Finally, we are developing new techniques such as noise spectral density measurements to enable us to probe physics not accessible by standard transport methods.
Previous research areas in our group include topological insulators, the anomalous Hall effect in ferromagnets, materials with large thermopower, unusual magnetism, charge density wave materials, and topics in biophysics.
Information about previous research areas may be found at our old website.